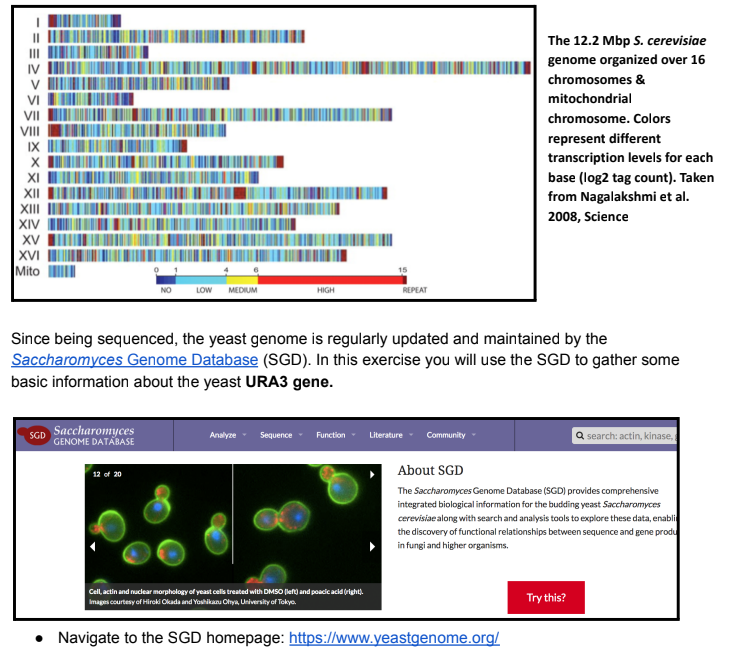
Yeast Mutagenesis Analysis for URA3 GENE
Data mining the Saccharomyces Database(SGD) to analyze Subcellular Localization/biochemical functions of stop codons compromising cDNA through EMBOSS Transeq.
Yeast Mutagenesis Analysis for the URA3 Gene
Courtney Moulton, Alanna Eisenbeiser, Ramsey Hammad
4/19/2020
Procedures
Bioinformatics Analysis of the Yeast URA3 Gene
Genetics Lab
1. Data mining the Saccharomyces Genome Database (SGD)
Saccharomyces cerevisiae (S. cerevisiae) is more commonly known as baker’s yeast and has
been an important organism for baking, winemaking, and brewing since ancient times. However, as
we’ve discussed in lab, S. cerevisiae is also a powerful model organism for studying eukaryotic
genetics and genomics. It had the distinction of being the 1st eukaryotic genome to be fully
sequenced in 1996. The S. cerevisiae genome is ~12.2 megabase pairs (Mbp; millions of bp) and is
organized on 16 chromosomes as well as its small mitochondrial genome.
● Type “URA3” in the search window and navigate to the URA3/YEL021W gene index page
2. Obtaining and Translating cDNA Sequence for Protein Analysis
The Central Dogma of Molecular Biology was eloquently theorized by Francis Crick in 1956. This
fundamental theory explains the flow of genetic information within biological systems where DNA
genes are transcribed into RNA intermediate molecules, some of which can be translated into
proteins; or as Crick more simply put it “DNA makes RNA, makes proteins”.
The central dogma dictates that if we know the sequence of a protein coding gene (DNA), we can
deduce that protein’s amino acid sequence. To determine the amino acid sequence of the URA3
protein product you 1st need to obtain the URA3 coding sequence from the SGD URA3 index page.
From the URA3 SGD page, download the protein coding cDNA portion of the URA3 gene from SGD
for downstream analysis of the URA3 protein product
● Scroll back up to the “Sequence” portion of the index page and click the “Download (.fsa)” icon
● Select the “Coding DNA” option and open the downloaded file in MS Word or similar text editor
● Save the text file to your desktop as “wt_URA3_cDNA”
The text file you’ve downloaded from SGD is the cDNA or protein coding region of the URA3 gene.
This represents the mRNA sequence that is transcribed from the URA3 gene, which serves as the
template for protein translation of the URA3 protein product. The sequence is in FASTA format, a
text-based format for representing either nucleotide or peptide sequences using single-letter codes. A
sequence in FASTA format begins with a single-line description denoted by the “>” icon, followed by
lines of sequence data. Since it is a simple text file, it can be opened & edited on any computer.
Now that you have the URA3 coding sequence, you will use an online tool hosted by the European
Bioinformatics Institute (EBI) to virtually translate your cDNA sequence into a protein amino acid
sequence.
● Navigate to EBI EMBOSS Transeq web browser: https://bit.ly/2BgXvmD
● Copy/paste your FASTA formatted URA3 cDNA sequence into the DNA/RNA window
○ The “<” descriptor line should be included in the window to denote the start of a
sequence
● Keep all other default settings and hit submit
● After a few minutes you will see your translated amino acid sequence
Yeast Bioinformatics: Part 1
● Select the “Show Colors” output options to view your color coded translated sequence
○ Note: single letter amino acid codes are used in this output;
EMBOSS Transeq uses the following color code to indicate the biochemical properties of each amino
acid comprising the protein. The * icon indicates a stop codon was encountered in the cDNA:
The UniProt Database is a one stop shop protein knowledgebase that warehouses tons of useful
information about all characterized proteins. We will use this database to extract useful information
about the URA3 protein product, which you have hopefully figured out by now is called Orotidine
5'-phosphate decarboxylase or OMP decarboxylase for short.
● Navigate to UniProt (https://www.uniprot.org/) and search for “URA3”
● Lots of URA3 hits come up, click on entry for the S. cerevisiae OMP decarboxylase protein
The UniProt S. cerevisiae OMP decarboxylase page is a long entry detailing all of the known
information about the protein. The entry can be scrolled down or tabs on the left side can be clicked
to automatically jump to specific sections. For example, jump to the section detailing where OMP
decarboxylase is found in yeast cells.
● Click on the “Subcellular Localization” tab on the left navigation pane
○ Cellular areas/organelles highlighted in yellow indicate where the protein is expressed
● Next jump to the “Function” section to determine the biochemical function of OMP
decarboxylase (FYI, uridine monophosphate or UMP is the monomeric unit of the pyrimidine
RNA nucleotide uracil)
● Click on the “Feature Table” link under the “Display” options on the left panel to navigate to
several tables describing functionalities and characterized mutations in the OMP
decarboxylase protein
○ This page also has a numbered OMP decarboxylase amino acid sequence for reference
Results
To induce mutations, 1x10^8 cells/mL were placed onto a 5-FOA plate with uracil and the plate
was exposed to UV irradiation. To ensure that the mutants are 5-FOA resistant, we streaked out
colonies onto a uracil dropout plate, a YPD rich media plate and a 5-FOA plate with uracil. On
the 5-FOA plates that contained uracil, the wildtype yeast did not grow any colonies, while the
URA3 strain and mutants 154, and 156 all grew large colonies, and the mutant 155 strain grew
medium colonies (Table 1). On the dropout plates, the URA3 sample did not grow colonies and
neither did any of the mutants (Table 1). But when looking at the rich media, all strains of yeast
grew large colonies of yeast (Table 1). Scoring was based on presence or absence of streaks of
yeast seen on the plates, and how much was seen.
Complementation tests between the 5-FOA resistant mutants and known uracil mutant genes
were crossed on a uracil dropout plate and a YPD plate carrying rich media. The uracil dropout
plate showed that the wildtype strain was present with each known mutant, while the URA3
sample did not grow where the URA3 mutant was spread, and mutants 154, 155, and 156 did not
grow where the URA3 mutation was spread (Fig.1). On the rich media plate, the 154, 155, and
156 mutants grew where each of the known uracil mutants were spread except for on the URA3
mutant (Fig. 2).
We extracted DNA from the three mutant strains of yeast and used the products for PCR, which
allowed us to run gel electrophoresis (Fig. 3). The marker was just over 1 Kb and the wildtype
yeast had a band that was about 950 bp. There were no bands in lane 2 which contained mutant
154, no bands in lane 3 showing mutant 155, and no bands in lane 4 with mutant 156, or lane 5
which contained no DNA and acted as a control. Given that no bands were present, to continue
the experiment we used mutant 23 which was known to have amplified DNA that could be sent
for sequencing.
DNA subway analysis was used to examine the DNA structure of mutant 23. There was a change
in nucleotides in mutant 23 compared to the consensus sequence around base pair 272,
substituting a cytosine (C) for a guanine (G) (Fig. 4).
The protein coding region of the URA3 gene was used to be translated into the protein amino
acid sequence (Fig. 5). Analysis of the protein structure using EMBOSS Transeq showed that
there was a change in amino acid from protein D in the wildtype yeast to protein E in the mutant
yeast (Fig. 6). The colors are representative of properties of the protein and the change in the
protein did not change the property of the protein; therefore, E and D are both acidic in nature
(Fig. 7). Analysis of the mutant showed the resulting amino acid from the nucleotides had
changed from Aspartic acid to Glutamic acid (Fig. 8). We used the YASARA program to
visualize this change in amino acid and found that the amino acid had changed at position 91 and
the change was very small and hard to see (Fig. 9).
Discussion
UV mutagenesis was used for the generation of auxotrophic mutants. Using the UV light allows
the yeast strains to be exposed to a mutagen thus making it more likely for the cells to mutate.
The mutants were selected in the presence of 5-FOA (5-Fluoroorotic acid), uracil, and rich
plates. During our experiment we had labeled our plates in accordance with the lab manual
designating our rich media plates with a blue stripe, the 5-FOA plates with a red stripe, and our
uracil drop out plates with two black stripes in order to lower the probability of experimental
errors to which none had arose. In order to score our results, we had based scoring off of the
presence or absence of yeast on our plates and how much was seen. The 5-FOA plates indicate
yeast cells that are resistant to 5-FOA which could cause mutations within the URA3 gene. This
effect is because when yeast cells are grown on the 5-FOA compound the enzyme orotidine
5’-phosphate decarboxylase, which is encoded by the URA3 gene, is unable to recognize its
normal substrate. This causes the enzyme to convert the substrate into uracil and it will convert
5-FOA into a toxic product, 5-fluorouracil, that can kill the cell (Hamedi et al., 2013). The uracil
auxotrophic phenotype was confirmed by the ability of mutants to grow in the presence of uracil
and the lack of growth in the absence of this compound.
It was also concluded that all the samples produced large colonies on the rich media plates which
was predicted considering that it contains an excess of nutrients making it an excellent source of
nutrition for any yeast strain and confirms that our mutants were able to survive and/or grow.
While the wildtype yeast produced a strong growth it had proved to be the opposite on our
5-FOA with uracil plates to which the wildtype yeast showed no growth of any colonies. Mutant
154 and mutant 156 both showed strong growth of large colonies in our 5-FOA with uracil plates
while mutant 155 was recorded to have had a medium growth in accordance when being exposed
to the same plate. This was expected because the yeast cells survived meaning that they could
have mutations within the URA3 gene. As we had expected, there was no growth in the uracil
drop-out plates or in any of our mutants which was supported by our data.
To test whether the uracil auxotroph phenotype is due to the inactivation of the URA3 gene, a
complementation test was completed. Complementation tests are used to confirm from which
gene the mutation arose. Known uracil mutant genes and the resistant 5-FOA mutants were
crossed onto a YPD-rich media plate and a uracil dropout plate and showed that the mutations
were on the URA3 gene because mutants 154, 155, and 156 did not grow where the URA3
mutant was streaked on either plate (Fig. 1-2). We know that the known mutant URA3 sample is
unable to make uracil due to the fact that it is unable to produce the enzyme orotidine
5’-phosphate decarboxylase, the enzyme that is encoded by the URA3 gene. The mutants we
worked with did not compensate for the missing enzyme in the URA3 mutant so we can
conclude that mutants 154, 155, and 156 were unable to make the enzyme orotidine 5’-phosphate
decarboxylase, meaning that the mutations were most likely on the URA3 gene.
To compare the DNA of the mutant and the wildtype yeast, DNA was extracted from the mutants
and DNA was amplified through PCR. To ensure that there were DNA samples to be sent for
sequencing, we ran gel electrophoresis. The gel electrophoresis showed us that our mutants did
not show up which was not expected (Fig. 3). The error was not due to contamination as the
control did not show a band in the gel so there could have been human error in the preparation of
the PCR products, or error in extracting DNA from the yeast. To continue the analysis of a
URA3 mutant, mutant 23 was provided and had known DNA that was amplified.
Given the results of the DNA changes in the URA3 mutant, the protein structure of the wild type
and mutant 23 yeast samples were analyzed. Our mutation was around position 272 and in the
URA3 gene there is a binding site at position 235 which acts as a substrate; therefore, our
mutation could have changed the way a protein binds to that substrate or might not be able to
bind at all (Rose et al., 1984). DNA subway showed that there was one nucleotide difference
between the wildtype and mutant yeast strands from a cytosine to a guanine so we know that our
protein structures could be different (Fig. 4). Using the EMBOSS program, we were able to see
the protein sequences for the mutant and wildtype yeast and found one difference between them
(Fig. 6). The change in the protein in the mutant strain was found to be acidic like the wild type
strain, meaning that it will still have the same properties as the wildtype yeast, and they will both
be hydrophilic in water (Fig. 7). The amino acid change in the mutant was due to a point
missense mutation because there was only a single nucleotide change from the wildtype to the
mutant yeast. Looking at the structure of the amino acid change from an Aspartic acid to a
Glutamic acid, they look relatively similar, except that the tails almost mirror each other (Fig. 8).
To visualize this, we were able to use YASARA to see the minor change from the wildtype
protein and the mutant protein (Fig. 9). We can conclude that given even a slight change to a
nucleotide can alter an amino acid that codes for a protein and thus breaks the functional URA3
gene, making it unable to produce uracil on its own.
Given that we were able to make yeast mutants and continue the analysis of the mutation to find
where in the gene the mutation had occurred, we can conclude that our hypothesis was supported
that the mutations we generated were due to changes in the URA3 gene.
Due to the fact that UV light is a mutagen that can damage DNA in our skin cells and give rise to
mutant cells that grow uncontrollably as cancerous tumors, the biological implications of this
experiment can be pertained to the testing of sunscreens and related skin care products in their
ability to block the sun's harmful UV rays (Xing et al., 2011). The data that was collected is also
useful to see how radiation could possibly affect skin cells and how certain mutations can also
lead to cancer or cell death. In terms of the application of what we learned onto future
experiments we had discovered the importance of complementation tests. One study found that
when using this mutagenesis procedure on probiotic yeast, it was concluded that the yeast has
acid and bile resistant properties that could have practical implications as pharmaceuticals that
can be used on the GI tract (Hamedi et al., 2013).
References
Hamedi, H., Misaghi, A., Modarressi, M. H., Salehi, T. Z., Khorasanizadeh, D., Khalaj, V.
(2013). Generation of a uracil auxotroph strain of the probiotic yeast Saccharomyces
boulardii a host for the recombinant protein production. Avicenna Journal of Medical
Biotechnology, 5(1) pp. 29-34.
https://eds.a.ebscohost.com/eds/pdfviewer/pdfviewer?vid=8&sid=2af3d46e-a572-46e1-b
60f-9e3e9a8d3379%40pdc-v-sessmgr06
Rose, M., Grisafi, P., Botstein, D. (1984). Structure and function of the yeast URA3 gene:
expression in Escherichia coli. Gene, 29(1-2) pp. 113-114.
https://www.sciencedirect.com/science/article/abs/pii/0378111984901720?via%3Dihub
DOI: 10.1016/0378-1119(84)90172-0
Xing, J.Y., Bai B., Chen, Z. H. (2011). Effect of UV irradiation on stabilization of collagen.
International Symposium on Water Resource and Environmental Protection, 4, pp.
2979-2982.
https://eds.a.ebscohost.com/eds/detail/detail?vid=16&sid=2af3d46e-a572-46e1-b60f-9e3
e9a8d3379%40pdc-v-sessmgr06&bdata=JkF1dGhUeXBlPWNvb2tpZSxpcCxhdGhlbnM
sc2hpYiZzaXRlPWVkcy1saXZlJnNjb3BlPXNpdGU%3d#AN=edseee.5893503&db=eds
eee
Power in Numbers
Project Gallery
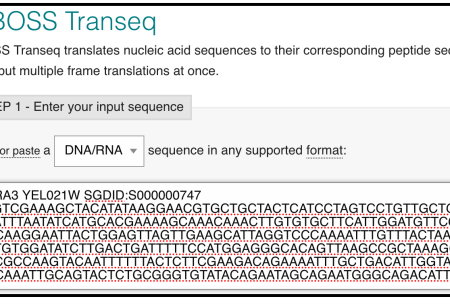

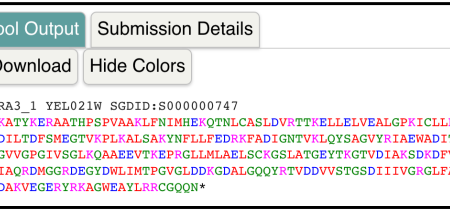

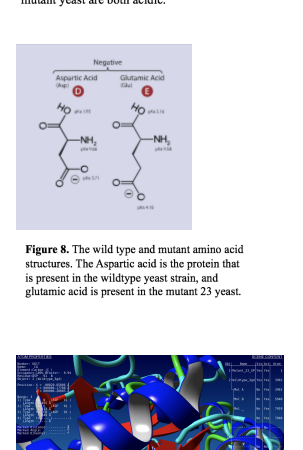
